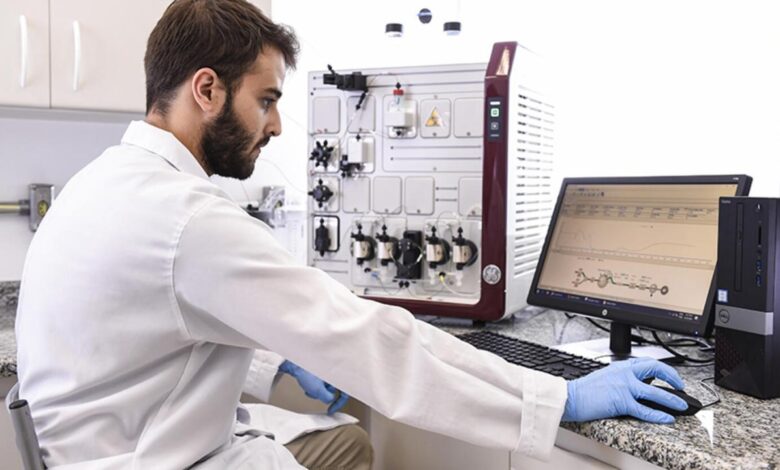
Bioterrorists Trick COVID-19 Vaccine Developers with Malware
Bioterrorists to trick covid 19 vaccine developers with malware – Bioterrorists trick COVID-19 vaccine developers with malware – a chilling thought, right? This isn’t some far-fetched sci-fi plot; it’s a very real and terrifying possibility. Imagine the devastating consequences if malicious actors successfully infiltrated the crucial process of vaccine development, potentially delaying or even sabotaging the creation of life-saving treatments. This post delves into the methods, motivations, and potential impacts of such an attack, exploring the vulnerabilities within the system and the crucial defenses we need to put in place.
We’ll explore how cyberattacks could manipulate research data, steal intellectual property, or even introduce false results into clinical trials. The implications extend far beyond scientific setbacks; a successful attack could have catastrophic effects on global health, the economy, and international stability. We’ll examine the different stages of vaccine development most susceptible to these attacks and discuss the potential defensive strategies to mitigate this threat.
Get ready to dive into a world where the fight against disease intersects with the fight against cybercrime.
Methods of Malware Delivery
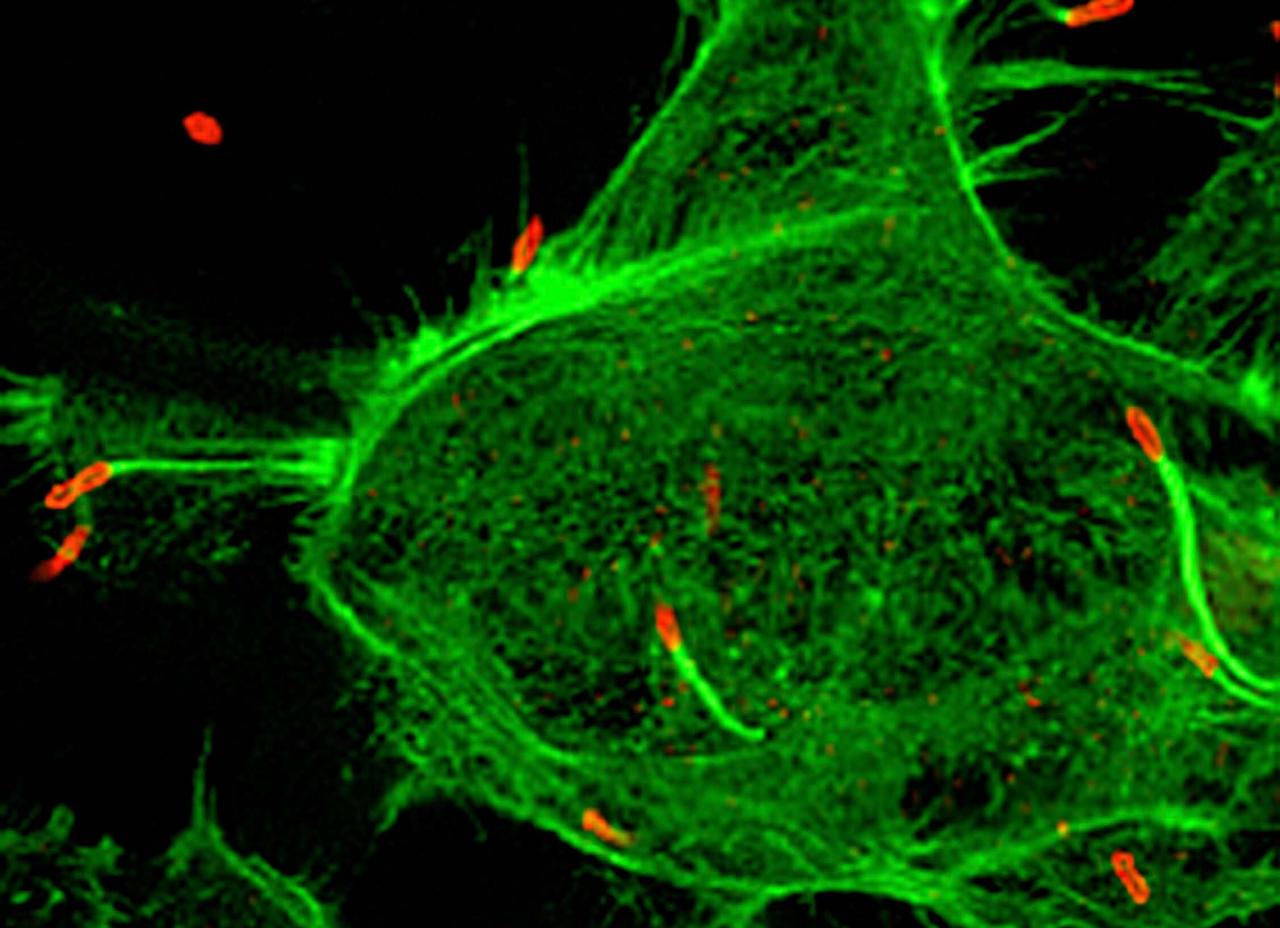
Targeting vaccine developers with malware is a sophisticated undertaking, requiring a deep understanding of their workflows and security practices. Bioterrorists would likely leverage several methods, each with its own strengths and weaknesses, to maximize their chances of success. The choice of delivery method would depend on factors such as the target’s technological sophistication, their security protocols, and the bioterrorist’s resources.
Phishing Emails
Phishing remains a highly effective method for delivering malware. Bioterrorists could craft convincing emails mimicking legitimate communications from colleagues, research institutions, or funding agencies. These emails might contain malicious attachments (e.g., Word documents with embedded macros, PDFs with JavaScript exploits) or links to compromised websites hosting malware. The vulnerability exploited here is human error – a user’s trust and lack of caution in opening suspicious emails or clicking links.
A successful phishing campaign relies on social engineering to manipulate the recipient into taking the desired action. For example, an email might claim to contain urgent research data or a grant application update, compelling the recipient to open the malicious attachment.
Infected USB Drives
Leaving infected USB drives in easily accessible locations (parking lots, near building entrances) is a more passive approach. The vulnerability lies in the user’s lack of awareness and the inherent trust placed on physical media. Upon insertion into a computer, the malware can automatically execute, exploiting operating system vulnerabilities or using auto-run features. The success rate is lower than targeted phishing, but it requires less technical expertise and offers a degree of anonymity.
A real-world example of this method would be leaving a seemingly innocuous USB drive labeled “Research Data” near a research facility.
Compromised Software Updates
Bioterrorists could infiltrate legitimate software update channels or create fake update sites. The vulnerability here is the reliance on automatic updates and the trust placed in the software provider. By replacing genuine updates with malicious code, the attackers can gain access to the target’s system without raising immediate suspicion. This method is particularly effective because updates are often downloaded and installed without much scrutiny.
Imagine a scenario where a bioterrorist compromises a widely used scientific software’s update server, replacing the legitimate update with malware that steals sensitive data or disrupts research operations.
Table Comparing Malware Delivery Methods
Method | Stealth | Success Rate | Detection Difficulty |
---|---|---|---|
Phishing Emails | Moderate (depends on sophistication) | Moderate to High (depends on social engineering) | Moderate (anti-spam filters and security software can help) |
Infected USB Drives | High (if left discreetly) | Low (relies on chance discovery) | High (detection often relies on post-infection analysis) |
Compromised Software Updates | High (mimics legitimate updates) | High (if successful infiltration) | High (requires sophisticated analysis to detect altered updates) |
Malware Functionality and Objectives
Disrupting the development of a COVID-19 vaccine through cyberattacks presents a chillingly effective method of bioterrorism. The sophistication of such an attack hinges on the malware’s ability to subtly infiltrate systems, manipulate data, and ultimately hinder the progress of vital research. This requires a multi-pronged approach, utilizing various malware types to achieve specific objectives.Malware employed in this scenario wouldn’t necessarily aim for immediate, catastrophic system failure.
Instead, the goal would be insidious, long-term disruption, maximizing damage while remaining undetected for as long as possible. This necessitates the use of sophisticated, stealthy malware capable of evading detection and performing its malicious tasks without raising alarms.
Types of Malware Used to Disrupt Vaccine Development
Several malware types could be effectively deployed to achieve the desired disruption. These include advanced persistent threats (APTs), designed for long-term, covert data exfiltration and manipulation; rootkits, providing persistent, hidden access to compromised systems; and ransomware, although its immediate crippling effect might be counterproductive in a long-term bioterrorism strategy, could be used selectively to target specific, high-value research groups or facilities.
Data-altering malware, specifically designed to subtly corrupt research data without obvious signs of tampering, would be crucial. Finally, spyware could be used to monitor research progress and identify vulnerabilities to be exploited.
Examples of Malware Impact on Vaccine Development, Bioterrorists to trick covid 19 vaccine developers with malware
The impact of such malware could be devastating. Imagine a scenario where an APT gains access to a research institution’s database. It could subtly alter experimental results, introducing minor inaccuracies that accumulate over time, leading to flawed conclusions and wasted resources. Alternatively, the malware could steal intellectual property, giving competitors an unfair advantage or even allowing for the development of a less effective or even dangerous vaccine.
Data exfiltration could delay the process by forcing researchers to re-collect and re-analyze data, costing valuable time and resources.
Malware Causing False Research Results or Compromising Vaccine Trials
The potential for malware to create false research results is particularly alarming. A sophisticated piece of malware could systematically introduce biases into data sets, leading to inaccurate conclusions about vaccine efficacy and safety. This could compromise the integrity of clinical trials, potentially delaying or even preventing the approval of a safe and effective vaccine. For instance, malware could manipulate data showing adverse effects, creating a false impression of vaccine danger, leading to public distrust and hindering vaccination campaigns.
Conversely, it could falsely inflate efficacy rates, leading to premature deployment of an unsafe vaccine. Both scenarios have catastrophic consequences.
Hypothetical Malware Program: “Project Chimera”
Let’s consider a hypothetical malware program, codenamed “Project Chimera.” This APT-style malware would initially infiltrate systems through spear-phishing emails targeting key researchers. Once inside, it would establish persistent access using a rootkit, remaining undetected. Its primary function would be the subtle alteration of research data. “Project Chimera” would analyze research data in real-time, identifying statistically insignificant outliers.
It would then subtly modify these outliers to create a pattern suggesting a negative effect (e.g., increased adverse reactions) or decreased efficacy. This manipulation would be gradual and carefully calibrated to avoid immediate detection. Simultaneously, a spyware component would monitor communication and research progress, identifying any attempts to detect the malware’s activity. The data alteration would be designed to subtly delay the vaccine development process, possibly by years, without causing a catastrophic system failure that would trigger an immediate investigation.
The overall effect would be a prolonged and ultimately unsuccessful vaccine development effort, achieved through a slow, insidious erosion of trust in the research. The success of Project Chimera relies on its stealth and the gradual, incremental nature of its data manipulation. This allows it to remain undetected while gradually undermining the entire research process.
Targeting Vaccine Development Stages
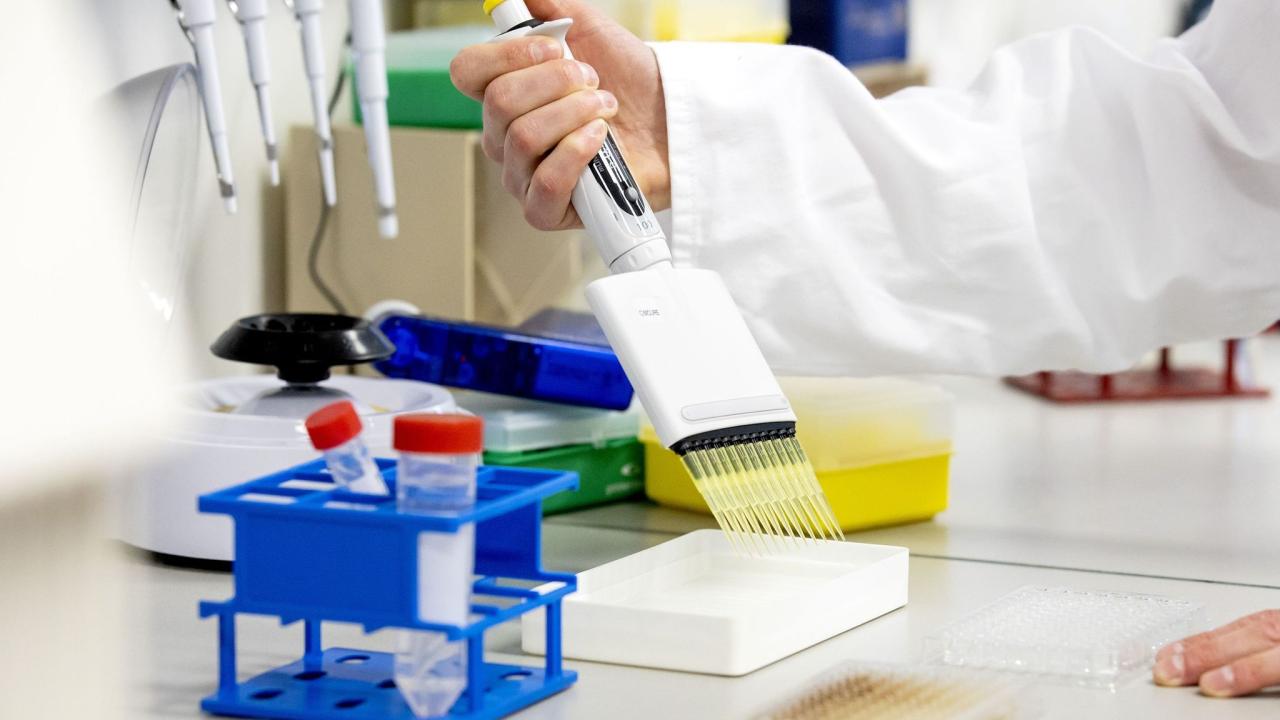
The development of a vaccine is a complex, multi-stage process, each step offering potential vulnerabilities exploitable by sophisticated malware. A successful attack at any stage could significantly delay or even derail the entire project, with potentially devastating consequences for public health. This analysis focuses on the critical stages most susceptible to malicious cyberattacks and the specific ways malware could be deployed to disrupt them.Targeting these stages requires a deep understanding of the scientific processes involved and the digital infrastructure supporting them.
The sophisticated nature of the attacks necessitates a multi-pronged approach, leveraging various malware techniques to achieve maximum disruption.
Research Stage Targeting
The initial research phase, involving the identification of potential vaccine candidates and the initial testing of their efficacy, is particularly vulnerable. Malware could be introduced through compromised email attachments containing malicious macros or through infected software updates targeting laboratory equipment. This could lead to the alteration or deletion of crucial research data, slowing down or completely halting the research process.
For example, a sophisticated piece of malware could subtly alter the results of in-vitro experiments, leading researchers down a false path and wasting valuable time and resources. The consequences include delays in vaccine development and the potential loss of promising vaccine candidates.
Pre-Clinical Trials Targeting
Pre-clinical trials, involving animal testing to assess safety and efficacy, also present vulnerabilities. Malware could be used to manipulate the data collected from these trials, leading to inaccurate results that could affect the decision to proceed to human clinical trials. Imagine malware subtly altering the recorded health metrics of test animals, either exaggerating positive results to push a flawed vaccine forward or underrepresenting efficacy to hinder a promising candidate.
The consequences of such manipulation could range from wasted resources to the release of an ineffective or unsafe vaccine.
Clinical Trials Targeting
Clinical trials, involving human subjects, are exceptionally sensitive to data manipulation. Malware could be used to alter patient data, potentially leading to inaccurate assessments of vaccine efficacy and safety. For instance, malware could systematically delete or modify adverse event reports, leading to an underestimation of potential risks. This could have catastrophic consequences, potentially resulting in the approval and widespread distribution of a dangerous vaccine.
The potential for harm extends beyond immediate health risks, as compromised trial data could erode public trust in the entire vaccine development process.
Manufacturing Stage Targeting
The manufacturing stage, where the vaccine is produced at scale, is susceptible to disruption through attacks targeting the manufacturing processes and supply chains. Malware could be deployed to disrupt production lines, corrupt quality control data, or even introduce contaminants into the vaccine itself. Consider a scenario where malware alters the manufacturing parameters, resulting in a batch of vaccines with reduced efficacy or increased risk of adverse events.
This would lead to significant delays, financial losses, and potential harm to those who receive the affected vaccines.
Potential Impact of Successful Malware Attacks
The following list summarizes the potential impact of successful malware attacks at each stage:
- Research Stage: Delays in vaccine development, loss of promising vaccine candidates, wasted resources, compromised research integrity.
- Pre-Clinical Trials: Inaccurate assessment of vaccine safety and efficacy, flawed decision-making regarding clinical trials, wasted resources.
- Clinical Trials: Inaccurate assessment of vaccine safety and efficacy, potential approval of an unsafe or ineffective vaccine, erosion of public trust.
- Manufacturing Stage: Disruption of production lines, compromised quality control, potential contamination of vaccines, delays in vaccine distribution, significant financial losses.
Defense Strategies and Mitigation Techniques
Protecting the sensitive research data involved in COVID-19 vaccine development from bioterrorist attacks requires a multi-layered security approach. This isn’t just about preventing initial infection; it’s about building resilience to withstand and recover from a successful attack. A robust strategy combines technological safeguards with stringent operational procedures and a culture of security awareness.The threat landscape is constantly evolving, so a proactive and adaptable security posture is crucial.
This involves regularly updating security protocols, conducting penetration testing to identify vulnerabilities, and providing ongoing training for researchers and staff on best security practices. A layered approach ensures that if one defense fails, others are in place to mitigate the damage.
Network Security Measures
Implementing a robust network security infrastructure is paramount. This includes deploying firewalls to control network traffic, intrusion detection and prevention systems (IDPS) to monitor for malicious activity and block suspicious connections, and virtual private networks (VPNs) to encrypt data transmitted over public networks. Regular patching of operating systems and software is essential to close known vulnerabilities exploited by malware.
Network segmentation can further limit the impact of a breach by isolating sensitive systems from the rest of the network. For example, isolating the servers containing vaccine development data from the general research network would prevent a compromise of one area from automatically impacting the other. Furthermore, employing advanced threat protection solutions, like sandboxing, allows suspicious files to be analyzed in a controlled environment before they can execute on the network.
Endpoint Security
Protecting individual workstations and servers is crucial. This involves deploying endpoint detection and response (EDR) solutions that continuously monitor system activity for malicious behavior. Antivirus and anti-malware software should be installed and kept up-to-date on all devices. Regular security scans should be performed to detect vulnerabilities and malware. Restricting administrative privileges to only authorized personnel helps limit the damage a compromised account can cause.
Data Loss Prevention (DLP) tools can monitor and prevent sensitive data from leaving the network without authorization. A good example of DLP in action would be preventing a researcher from emailing a large file containing sensitive vaccine sequence data to a personal email account.
Data Backup and Recovery Systems
Robust data backup and recovery systems are vital to minimize the impact of a successful attack. A multi-layered backup strategy, incorporating both on-site and off-site backups, is recommended. Regular backups should be performed, and the backups should be tested regularly to ensure they are recoverable. This ensures that even if data is lost or corrupted in an attack, it can be restored quickly and efficiently.
Consider using immutable backups, which cannot be altered or deleted, to protect against ransomware attacks. The implementation of a comprehensive disaster recovery plan should also be in place, detailing how to restore operations in the event of a major disruption. This plan should include the restoration of systems, data, and business continuity procedures.
The thought of bioterrorists using malware to infiltrate COVID-19 vaccine development pipelines is chilling. Imagine the sophisticated attacks they could launch, potentially compromising years of research. To counter such threats, robust and secure development platforms are crucial; that’s where learning about domino app dev the low code and pro code future becomes vital for building secure systems.
Ultimately, strengthening our digital defenses is paramount to preventing these kinds of bioterrorism scenarios.
Best Practices for Securing Sensitive Research Data
The following best practices are essential for securing sensitive research data and preventing malware infiltration:
- Implement strong access controls, including multi-factor authentication (MFA), to restrict access to sensitive data.
- Enforce strong password policies and regularly change passwords.
- Educate researchers and staff on security awareness and best practices, including phishing awareness training.
- Regularly conduct security audits and penetration testing to identify vulnerabilities.
- Use encryption to protect data both in transit and at rest.
- Segment the network to isolate sensitive systems and data.
- Implement a robust incident response plan to handle security incidents effectively.
- Regularly update software and operating systems to patch security vulnerabilities.
- Use version control systems to track changes to research data and enable rollback to previous versions if necessary.
- Conduct thorough background checks on all personnel with access to sensitive data.
Impact and Consequences
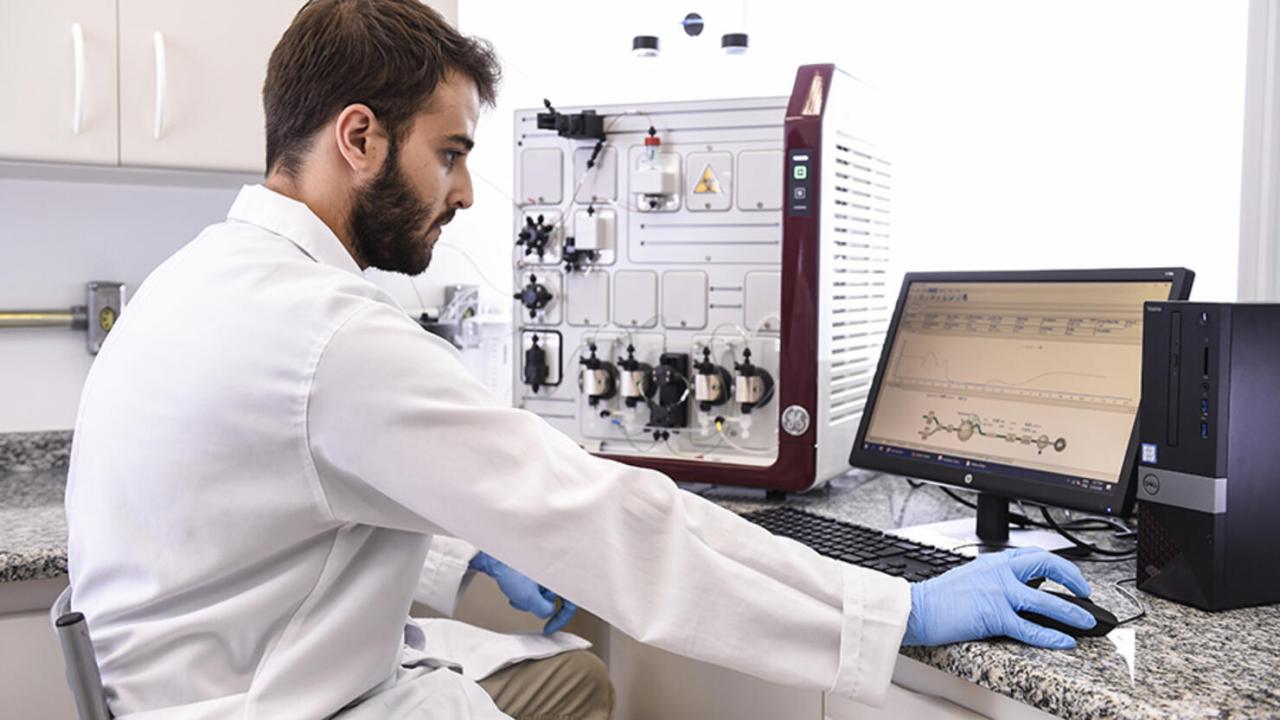
A successful bioterrorist attack leveraging malware to compromise vaccine development would have devastating and far-reaching consequences, extending far beyond the immediate disruption of a single vaccine program. The ripple effects would be felt globally, impacting public health, the economy, and international relations in profound ways. The scale of the damage would depend on the stage of vaccine development targeted, the sophistication of the malware, and the effectiveness of the response.The consequences of such an attack would be multifaceted and deeply interconnected.
The most immediate impact would be the delay or complete failure of the targeted vaccine development program, potentially leaving populations vulnerable to a deadly pathogen for an extended period. This delay could lead to a surge in infections, hospitalizations, and deaths, overwhelming healthcare systems and causing widespread panic. Beyond the direct health impacts, the economic consequences would be significant, with disruptions to global supply chains, decreased productivity, and increased healthcare costs.
The geopolitical ramifications could be equally severe, potentially exacerbating existing tensions and leading to international conflicts as nations compete for scarce resources and blame each other for the crisis.
Public Health Ramifications
The most obvious and immediate impact would be a significant increase in morbidity and mortality. A delay in vaccine development, even a relatively short one, could result in hundreds of thousands, or even millions, of additional infections and deaths, depending on the severity of the pathogen and the size of the vulnerable population. This would be especially true for vulnerable populations like the elderly and those with underlying health conditions.
Moreover, the disruption to vaccine development could erode public trust in scientific institutions and vaccination programs, leading to vaccine hesitancy and hindering future public health efforts. Imagine, for instance, a scenario similar to the 1918 influenza pandemic, where a delayed vaccine development exacerbated the already catastrophic situation, resulting in a prolonged period of suffering and death. The lack of a readily available and effective vaccine would lead to a prolonged period of social distancing measures, impacting education, the economy, and mental health.
Economic Impacts
The economic consequences of a successful attack would be widespread and severe. The immediate costs would include the increased healthcare expenditures associated with treating a larger number of infected individuals. Beyond direct healthcare costs, there would be significant losses in productivity due to illness, quarantine measures, and disruptions to essential services. The global economy would suffer a substantial blow, with major industries such as tourism, hospitality, and transportation experiencing significant declines.
The financial markets would likely react negatively, leading to increased volatility and potential economic recession. For example, the economic disruption caused by the COVID-19 pandemic provides a stark illustration of the potential magnitude of the economic damage. The pandemic’s impact extended beyond direct healthcare costs to encompass widespread business closures, job losses, and a global recession. A bioterrorist attack would likely amplify these effects.
Geopolitical Implications
A successful attack could trigger significant geopolitical instability. Nations might engage in blame games, potentially escalating existing tensions and leading to international conflicts. The competition for scarce resources, such as vaccines, personal protective equipment, and medical supplies, could exacerbate existing rivalries and create new ones. The loss of trust in international cooperation could hinder efforts to address the crisis collectively.
Furthermore, the attack could be used as a pretext for aggressive actions by states or non-state actors seeking to exploit the chaos and instability. Consider the heightened tensions during the early stages of the COVID-19 pandemic, where nations engaged in a race to secure limited supplies of medical equipment and vaccines. A deliberate attack would intensify these dynamics and potentially lead to more serious geopolitical consequences.
Final Summary
The threat of bioterrorists using malware to disrupt COVID-19 vaccine development is a stark reminder of the vulnerabilities within our critical infrastructure. While the potential consequences are immense, proactive measures can significantly reduce the risk. Strengthening cybersecurity protocols, implementing robust data protection strategies, and fostering international collaboration are crucial steps in safeguarding the future of vaccine development. This isn’t just about science; it’s about protecting global health and security.
The stakes are simply too high to ignore.
Key Questions Answered: Bioterrorists To Trick Covid 19 Vaccine Developers With Malware
What types of malware are most likely to be used in such attacks?
Advanced persistent threats (APTs), designed for stealthy data exfiltration and manipulation, are a prime concern. Ransomware could also be used to cripple research facilities. Trojans and backdoors would allow for long-term access and control.
How can vaccine developers detect these attacks?
Regular security audits, intrusion detection systems, and employee security training are essential. Careful monitoring of system logs and data integrity checks can also help identify anomalies.
What role does international cooperation play in preventing these attacks?
Sharing threat intelligence, coordinating cybersecurity best practices, and jointly developing countermeasures are crucial. International collaboration is vital to protect global health security.
What are the long-term implications of a successful attack?
Beyond immediate setbacks in vaccine development, a successful attack could erode public trust in vaccines, create vaccine hesitancy, and potentially lead to future outbreaks.